서론
대뇌피질의 전기자극은 다양한 신경학적 질환에 광범위하게 사용되고 있다. 특히 수술 중 대뇌피질에서 전기자극의 사용은 뇌지도화(brain mapping) 및 신경계 감시를 가능하게 하였다. 이러한 수술중신경계감시(intraoperative neurophysiological neuromonitoring, IONM)는 Galvani와 Volta 이후로 수세기 동안 과학과 의학의 발전을 통해 확립되었다.
1870년 Fitz와 Hitzing이 개의 대뇌피질에서 전기자극을 통해 운동영역의 기능을 기술하였고[1], 1874년 Bartholow가 처음으로 인간의 대뇌피질에서 전극을 삽입한 이후[2], 전기자극을 사용한 많은 연구를 통해 신경생리를 이해하게 되었다. 이러한 토대에서 1937년에는 Penfield와 Boldrey가 양극성 전기자극(bipolar electrical stimulation)을 사용하여 대뇌운동피질의 지도화(motor cortex mapping)를 보고하고[3], “Penfield Technique”이라 알려진 수술중직접피질자극법(intraoperative direct cortical stimulation)을 제시하였다[4]. 또한 Patton과 Amassian은 단일펄스피질자극(single pulse cortical stimulation)이 D 파형와 I 파형을 발생한다고 보고하였고[5], 1993년 Taniguchi 등이 마취상태에서 고빈도 단극성 피질자극(high frequency monopolar cortical stimulation)이 안정되게 근육 운동유발전위(muscle motor evoked potential)를 유발한다고 하여[6], 이후 대뇌피질의 전기자극을 활용한 IONM은 임상에서 유용하게 사용되고 있다.
하지만 전기자극에 의해 뇌조직이 손상될 수 있다는 것이 과학과 의학 모든 측면에서 중요한 문제로 제기되었다. 따라서 지난 반세기 동안 전기 자극의 방법, 길이, 강도 등 변수(parameter), 전극의 재질, 전기자극에 따른 조직학적 변화 등 안전성과 관련된 많은 연구가 보고되고 있다. 대체로 이러한 연구들은 안정성에 대한 문제로 인간보다는 실험동물을 기반으로 하였다.
그러나, 실험동물과 인간에서 해부학적 특징과 전기자극의 적용의 차이가 있어 전기자극의 조직학적 역치(histologic threshold)에 따른 안전 한계(safety limit)를 그대로 직접 인간에게 적용하는 것은 어렵다[7–9]. 게다가 우리는 전기자극으로 인한 뇌조직 손상 기전에 대해 명확히 이해하지 못하고 있다.
따라서, 우리는 문헌고찰을 통해 전기자극에 대한 대뇌피질의 영향에 논의하고자 한다.
본론
전류(current)는 전자(electron)의 이동으로 전자는 음전하를 가지고 있어 음전위에서 양전위로 흐르지만, 전통적으로 전류는 양전위에서 음전위로 흐르는 양전하의 흐름으로 표현한다[10]. 전기자극 이후 전류는 음극(cathode, negative electrode)에서 흡수되고, 양극(anode, positive electrode)으로 흘러나온다. 자극의 선량은 전극의 접촉면적, 전류(current), 지속시간(duration or pulse width, D)으로 정의된다. 전류 I(in amperes, A)는 전하(charge)의 비율, 즉 흐름이며, 전하 Q(in coulombs, C)는 전기의 양(amount of electricity)으로 Coulomb C는 정전류(constant current) 1A가 1초 동안 흘렀을 때 운반되는 전하의 양이다[11,12].
전류밀도(current density, in A/cm2)는 전극에서 단위 면적당 흐르는 전류를 의미하며, 즉 신체를 통해 전하의 밀도와 전류강도에 영향을 받는다[8]. 전류밀도는 가장자리 또는 지점에 집중되어 최대로 나타날 수 있다.
전하밀도(charge density, in microcoulumbs/cm2, μC/cm2)는 일정한 넓이에 존재하는 전하의 총량으로 전하를 전극의 면적으로 나눈 것으로 전하의 기능을 의미하고, 전극과 신경사이의 거리와 저항에 따라 급격히 감소한다[13–15]. 또한 상당 전하(charge per phase, in microcoulombs per phase, μC/ph)는 전기자극의 자극 한 주기의 절반인 상(phase)에 걸친 전류의 적분이다[14]. 그리고 자극의 전력(in watts, W)은 전류와 전압(in volt, V)을 곱한 것이며, 자극의 에너지(in joules, J)는 전력과 지속시간을 곱한 것으로 열이 발생한다[15,16].
이러한 전류의 흐름을 방해하는 정도를 물리량으로 나타낸 것이 전기저항(resistance)이다. 즉, 전기저항에 따라 전기자극 이후 흐르는 전류의 양이 달라질 수 있고, 대뇌피질의 자극의 정도도 다르다. 대뇌피질로 전기자극을 하는 경우에는 뇌척수액의 전기저항에 비해 회백질(gray matter)은 4–5배, 백질(white matter)은 2–3배로 차이가 있어 각각 전류의 흐름이 다르다[17,18]
따라서 대뇌피질에 적절한 자극이 되기 위해서는 저항의 변화에 따라 전압을 조절하여 일정한 전류가 흐르게 하는 정전류자극(constant-current stimulation)과 저항에 흐르는 전류를 조절하는 정전압자극(constant-voltage stimulation) 중 어느 방법이 적절한지 고려해야 한다. 회백질과 백질의 전기저항의 차이를 따르면 정전류자극을 우선적인 방법으로 고려해 볼 수 있지만, 자극이 전달되는 경로에 저항이 낮은 두개골이나 뇌척수액이 있는 경우, 전류가 단락(shunt)되어 정전류자극에 의한 전류의 상당량이 우회경로를 통해 흘러버려 목표한 대뇌피질에 충분한 전류를 전달하지 못한다. 즉, 경두개전기자극(transcranial electrical stimulation)의 경우 좀 더 안정적으로 전류를 전달할 수 있는 정전압자극이 선호되며, 주변 뇌척수액 등의 상태에 따라 직접피질자극(direct cortical stimulation)의 경우 정전류자극이 필요할 수도 있다[10,19].
수술중 전기자극(intraoperative cortical stimulation)에 의해 대뇌피질이 손상되었다는 보고는 없었고, 임상적으로 사용하기에 충분히 안전하지만, 적절한 예방적 조치를 취하지 않는다면 인간조직에 영향을 줄 수 있다. 전기자극으로 유발된 뇌조직의 손상은 흥분독성(excitotoxicity), 전기화학반응(electrochemical reaction), 열 침착(thermal deposition) 및 세포의 항상성(cellular homeostasis) 변화에 의해서 일어날 수 있다[13,15,16,18,20,21].
흥분독성은 과도한 자극(overstimulation)에 의해 대뇌피질의 손상을 유발하는 것으로, 전기화학반응과 같은 다른 원인보다 중요하다[14]. 대뇌피질의 장기간이고 지속적인 고주파 전기자극은 실험동물에서 조직학적으로 신경손상을 유발한다[13–15, 20–24]. 신경손상의 Mass action 이론의 관점에서 상당 전하는 신경이 흥분되는 총 용량을 결정하고, 전하밀도는 전극에 가까운 신경들의 흥분된 비율을 결정하여, 과도한 전하와 전하밀도가 자극되면 유도된 신경발화(neural firing)가 세포외 환경(extracellular environment)의 변화를 유발하여 신경손상이 나타난다[14,21,25,26]. 따라서 전하 및 전하밀도가 대뇌피질과 전극사이에서 발생하는 비가역적인 전기화학적 생성물의 양에 영향을 미칠 수 있으므로 전기자극에 의한 신경손상이 전기화학반응보다는 흥분독성의 Mass action의 효과로 설명될 수도 있다[25].
고양이 대뇌피질의 전극자극 연구에서 Pudenz 등은 전기자극시 상당 전하가 0.3 μC/ph 이상으로 초과하게 되면 점차 대뇌피질이 손상받게 되고, 상당 전하 3.0 μC/ph에서 손상 정도가 최대에 이른다고 하였다[22]. 그리고 Yuen 등은 20시간 이상 50-Hz 양상성 자극(biphasic stimulation)시 신경손상의 역치가 전하밀도 40 μC/cm2/ph에서 상당 전하 0.4 μC/ph라고 보고하였다[23]. 그 후 McCreery 등은 전하밀도 12 μC/cm2/ph에서 상당 전하 6 μC/ph로 자극시 조직이 손상된 반면, 전하밀도 10 μC/cm2/ph에서 상당 전하 5 μC/ph로 자극시 손상이 없었다고 기술하였다[14]. 이러한 실험동물연구에서는 전하와 전하밀도가 독립적으로 신경손상을 일으키기 보다는 함께 작용하여 증대시키는 것으로 보인다[14,25,27]. 쥐(rat) 대뇌피질의 전기자극 연구에서는 10–90분 동안의 전류밀도 143–286 μA/mm2와 500-Hz 단극성 pulse(monopolar pulse) 자극으로 전류 40–50 mA에서 조직학적 대뇌피질의 손상을 발생시켰다[21,28]. 이러한 실험동물의 연구에서 나타난 전기자극에 대한 안전범위를 Shannon은 재처리하여 공식으로 제시하였다[20].
D는 상당 전하밀도(charge density per phase)를 뜻하며, Q는 상당 전하를 의미한다. k는 손상을 일으키거나 일으키지 않는 전기자극 범위를 뜻한다. 이러한 Shannon의 공식은 제한된 자료에서 정리되었지만 단순하다는 장점이 있다(Fig. 1).
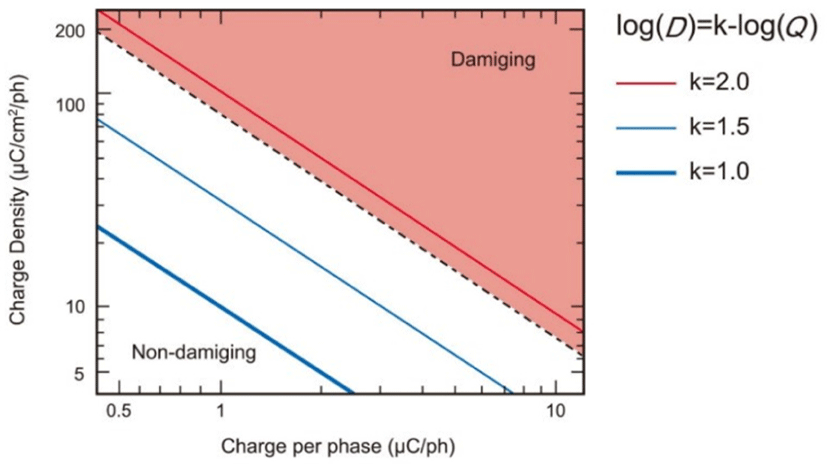
이러한 실험동물 연구를 통한 신경손상을 일으키는 전기자극의 역치가 수술 중 대뇌피질 자극에 유용한지는 여전히 명확하지 않다. Gordon 등은 상당 전하밀도 52–57 μC/cm2/ph와 상당전하 4.0–4.4 μC/ph에서도 인간 대뇌피질 자극시 어떠한 조직 손상이 없었다고 보고하였고[9], Taniguchi 등은 상당 전하밀도 12 μC/cm2/ph 미만에서 상당전하 6 μC/ph 이상에서도 임상적으로 인간 대뇌피질의 손상을 일으키지 않는다고 하였다[6,15]. 또한 다른 인간을 대상으로 하는 연구에서는 신경손상과 관련한 어떠한 임상 증상에 대한 보고는 아직 없다.
두번째의 전기자극에 따른 신경손상의 기전은 대뇌피질조직과 전극 사이의 전기화학반응이다[21,24,25,29–31].
두 금속 전극은 세포외액 같은 전해질에 놓여지고, 전해질과 금속사이에 접촉면이 이루어지며, 전류는 전해질을 통해 전극 사이로 흐르게 된다. 전해질에서 전하는 세포외액의 나트륨, 칼륨 및 염화물을 포함한 이온에 의해 운반된다. 따라서 전극과 전해질 사이의 접촉면에서 일어나는 주된 작용은 전극에서 운반되어진 전하를 전해질에서 이온으로 변환하는 것이다. 접촉면에서의 전하가 전달되는 주요 기전은 용량성반응(capacitive reaction)과 유도전류반응(faradaic reaction)이다. 평형(equilibrium)에 이르면 끌어당겨지거나 밀려난 이온을 통해 용량성전류가 흐른다. 평형을 이루지 못하면 산화-환원 과정을 통해 전극에서 전극으로 유도전류가 흐르게 된다. 용량성반응과 달리 유도전류반응은 전하가 평형에서 멀어질수록 비가역적인 전기화학적 생성물을 전해질에서 만든다. 금속이 심각하게 산화될 정도로 전극에서 전위가 초과하면 전극이 양극(anode) 처리되며, 전극은 부식되어 손상된다[25]. 즉, 세포외액 같은 염화물이 함유된 매질에서 백금(platinum)이 부식되는 것이 한 예이다. 전기자극은 안정상태에 도달할 때까지 점진적으로 전하를 평형에서 멀어지도록 전달하고, 전기화학적 생성물들을 대뇌피질 조직에 축적되게 할 수 있다. 이러한 독성의 정도는 중탄산염(bicarbonate), 인(phosphate)이나 세포내 단백질 등으로 완충될 수 있으며, 전기자극의 지속시간에 따라 달라진다.
1952년 Lilly 등은 전기화학반응으로 신경손상이 나타난다고 처음으로 보고하였는데, 이러한 개념은 자극 후 혈뇌장벽(blood-brain barrier) 파괴에 대한 여러 실험동물연구에서 확인되었다[24,29–32].
그러나 전기화학적 손상에 따른 임상적인 증상이나 징후는 여전히 보고되지 않았다. 흥분독성이나 전기화학반응으로 인간에서 신경손상에 관련한 어떠한 임상 증상에 대한 보고가 없는 것은 실험동물과 인간을 대상으로 하는 연구의 차이에서 비롯된다. 실험동물은 0.5–205시간 동안 연속적으로 전기자극을 받은 대신에 인간은 몇 초 동안 매우 짧고 간헐적으로 전기자극을 받았다. 지속적으로 전기자극을 받은 경우, 전기화학적 생성물이 신경손상을 일으킬 정도로 축적될 수 있으나, 간헐적인 전기자극의 경우 이 생성물이 손상을 일으키기 전에 주기적으로 완충될 수 있는 기회가 나타날 수 있다[33]. 그리고 간헐적인 전기자극은 금속 이온의 침착(deposition)되지 않을 정도로 전극의 분극(polarization)을 방지할 수 있다[33]. 또한 인간은 실험동물에 비해 더 두꺼운 연질뇌척수막(pia-arachnoid), 더 많은 뇌척수액 및 더 두꺼운 표재층을 가지고 있어 단락을 일으킬 수 있다[7,9,15,33]. 즉, 경두개전기자극(transcranial electrical stimulation)을 위해 발생하는 높은 전하는 두개골로 분산되고, 두피단락(scalp shunting)으로 인해 넓게 퍼진 부분만 대뇌피질로 통과된다[15,34](Table 1). 따라서 앞으로 이러한 보고가 없는 한 단상자극은 인간에게 안전하다고 여겨진다.
Stimulation type | Electrode diameter (mm) | Electrode material | Pulse duration (ms) | Intensity (mA) | frequency (Hz) | Charge/ph (μC/ph) | Charge density/pH (μC/cm2/ph) | Neural damage | |
---|---|---|---|---|---|---|---|---|---|
Experimental animal studies | |||||||||
Pudenz et al. 1977 [22] | Bipolar | 1.1, 1.4 | Pt, Rh | 0.1 to 0.5 | 3, 6 | 50 | 0.3 to 3.0 | 30 to 300 | + |
Agnew et al. 1977 [50] | 1.4 | Pt | 0.25 | 3 | 50 | + | |||
Yuen et al. 1981 [23] | 1.1 | Pt | 0.1 to 1.0 | 1.6 to 4 | 50 | 216 to 720 | 40 to 400 | + | |
Babb and Kupfer 1981 [27] | Bipolar | 0.2 | NiCr or Stainless steel | 0.1 | 0.5 to 2 | 2.5 to 240 | 0.4 to 14.4 | 11.11 to 66.6 | + |
Agnew et al. 1983 [51] | 1.1 | Pt | 0.25 | - | 20, 50 | - | 20, 100 | + | |
McCreepy et al. 1988 [52] | 1.1, 1.0 | Pt, Ta | 0.4 | > 2.5 | 50 | 0.8 to 1 | 80 to 100 | + | |
McCreepy et al. 1990 [53] | - | Pt | 0.4 | - | 50 | 0.05 to 18 | 10 to 1,600 | + | |
Oinuma et al. 2007 [28] | Monopolar | 1.0 | Ag | 0.2 | 1.5 to 50 | 500 | 0.3 to10 | 0.3 to 10 | + |
Intraoperative testing (clinical human studies) | |||||||||
Penfield and Jasper 1954 [4] | Bipolar | 1.0 | - | 2.0 | - | 60 | - | - | |
Ojemann 1979 [54] | Bipolar | 1.0 | - | 1.25 | 5 to 10 | 60 | 5.0 | 159 | |
Robertson 1979 [55] | 3.5 | Pt | 0.5,1.0 | - | 10 | - | - | + | |
Gordon et al. 1990 [9] | Bipolar | 3.175 | Stainless steel, 90%Pt–10%Ir | 0.3 | 12 to 15 | 50 | 4.4 | 5.7 | |
King and Schell 1987 [56] | Bipolar | - | - | 1.0 | 3 to 10 | 50 | 3 to 10 | 300 to 1,000 | |
Ojemann et al. 1989 [57] | Bipolar | 1.0 | - | 1.25 | 1.5 to 10 | 60 | 10.0 | 1,000 | |
Berger et al. 1990 [58] | Bipolar | 1.0 | - | 1.25 | 2 to 16 | 60 | 10.0 | 1,000 | |
Taniguchi et al. 1993 [6] | Monopolar | - | - | 0.4 | < 20 | 500 | <8 | <8 |
대뇌피질에 대한 전기자극은 또한 열에너지 소산으로 인해 열을 유발할 수 있다[16,21]. 따라서 최대강도에 가까운 고에너지 단-펄스 전기자극기(high energy short-pulse electrical stimulators)를 작동하는 경우, 열손상에 대한 주의가 필요하다[16]. 또한 국제전기기술위원회(International Electrotechnical Commission, IEC)에서는 열에 의한 손상을 피하기 위해 전기자극기가 1,000 Ω의 저항에서 펄스당 50 mJ을 초과해서는 안 된다고 한다[35]. 하지만 두피의 화상은 0.01% 발생율로 드물고, 자극으로 유발된 피부 및 신경의 열 손상의 보고는 아직 부족하다[16].
대뇌피질에 대한 전기자극은 세포의 항상성을 손상시키는 신경세포(neuron)의 과활성(neuronal hyperactivity)을 유도한다[18,21,23,26]. 전류가 신경세포에 흐르면 Ca2+의 세포내 농도와 K+의 세포내 농도가 증가한다. 이러한 변화로 인해 세포막과 신경세포가 파괴된다.
또한, 전기자극은 발작(seizure)과 후방전(afterdischarge)을 유발할 수 있으며, 실험동물연구에서 대부분 마취되지 않은 경우 발작과 후방전이 발생했다. 수초 동안 지속되는 50–60 Hz의 긴 펄스가 단일 펄스보다 발작과 후방전을 좀더 유발한다[15]. 그러나 이러한 발작은 전기자극에 따른 신경손상보다는 전기자극 자체로 인해 유발되었다[18,23,33,36,37]. 따라서 전기자극으로 유발된 발작은 뇌에 장기간 부정적인 영향을 주지 않을 것으로 생각되며, IONM 중 전기자극에 따른 발작은 부분발작(partial seizure)으로 쉽게 관리될 수 있다. 수술 중 전기자극에 의한 발작은 short acting barbiturate를 정맥주사하거나, 전기자극 중 cold Ringer’s lactate solution으로 세척(irrigation)하여 관리되며, 만약 의식변화를 동반한 발작이 발생하면 기능적인 수준으로 회복될 때까지 cortical mapping 과정을 일시적으로 중단할 수 있다[33]. 수술 중 간질의 위험을 줄이기 위해서는 후방전 역치보다 자극 강도를 높이지 않는 것이 좋다[33].
대뇌피질은 포유류의 대뇌 외층으로 중추신경계에서 가장 큰 통합영역이다. 피질영역은 각각 운동, 감각을 비롯해 지각, 인식, 생각, 기억, 언어, 의식 같은 고위피질기능을 담당한다. 대뇌피질에는 주요한 신경세포(neuron) 및 아교세포(glia cell)가 있다.
신경세포는 신경계의 구조 및 기능 단위이며, 신경자극(nerve impulse) 형태로 전기적 변화를 만들어내고, 시냅스(synapse)에서 화학적으로 전달한다. 아교세포는 신경세포 사이에 위치하여 신경세포접합부 및 신경세포의 신호전달능력을 유지하는 데 도움을 주지만, 신경세포와 달리 신경세포접합부의 상호작용 및 전기신호전달에는 관여하지 않는다.
전기자극에 의한 신경손상의 일반적인 조직학적 소견은 세포질 공포, 염색질 융해(chromatolysis)나 신경세포포식(neuronophagia)이며, 궁극적으로 신경세포가 완전히 소멸된다[9,22,28]. Pudenz 등은 고양이 대뇌피질의 양극성 전기자극에서 신경손상을 받으면 연질뇌척수막(leptomeninges)을 포함한 표재 대뇌피질 층에서 반응성 성상세포, 큰포식세포, 세포막의 불연속선, 부풀어오른 사립체, 팽창된 세포질그물과 글리코겐(glycogen) 침착 등 가장 현저한 조직학적 변화로 보고하였다[22]. Oinuma 등은 쥐(rat)의 대뇌피질에서 40–50 mA의 고빈도 단극성 전기자극(high-frequency monopolar electrical stimulation) 이후 수상돌기 및 사립체의 부종이 확인되었다고 기술하였다[28]. 저혈당과 kainic acid에 의한 간질발작 시 보여지는 현저한 세포내부종(intracellular edema)과 동반된 다염색질의 신경세포 위축(hyperchromic shrunken neuron), 수상돌기 부종과 유사하다[38–42]. 이 중 수상돌기의 부종은 수상돌기분지(dendritic arbor)에 따라 나타나는데, 수상돌기는 중추신경계의 신경세포 사이에서 흥분성 시냅스 입력(excitatory synaptic inputs)을 통합하는 데에 중요한 역할을 하므로 과도하게 시냅스가 활성화하는 상황에서 취약할 수 있어 전기자극으로 인한 신경손상의 또 다른 특징이다[28,38,39,43]. 그러나 저산소성 뇌손상에서 나타나는 세포외부종(extracellular edema)은 대뇌피질의 전기자극에 대한 선행연구에서는 관찰되지 않는다[44].
신경세포의 변화 외에도 중추신경계 손상에 따라 아교세포가 증식(proliferation)하고 비대(hypertrophy)해지는 아교증(gliosis)이 나타날 수 있는데, 아교세포는 시냅스 기능을 조절하는 세포외 이온과 신경전달물질의 농도를 유지하고 혈뇌장벽을 형성하는 데 관여한다[45]. 뇌에서 손상이 일어나면 큰포식세포와 아교세포가 손상 부위로 이동한 다음, 발생한 지 수시간 이내로 아교증이 시작되며, 3–5일 이후 재수초화(remyelination)에 기여하고, 마지막으로 아교세포 반흔(glial scar)을 형성한다[45,46]. 따라서 비활성 전극에서도 최소한의 대뇌피질 압박 및 이물(foreign body) 조직반응으로 피질층 Ⅰ, Ⅱ에서 경도 미만성 아교증과 경도 단핵 침윤(mononuclear infiltration)이 관찰된다[9,23,24,47]. Yuen 등은 Pulse duration (0.1, 0.25 및 1.0 ms), 자극 시간(1.5, 5, 6, 15, 20 및 50 hour) 및 전하밀도(40, 100 및 400 μC/ cm2/ph)를 각각 달리하여 고양이의 대뇌피질에 전기자극을 하고, 신경손상에 대한 조직학적 평가를 하였다[23]. 40 μC/ cm2/ph의 전하밀도에서는 피질층 Ⅰ에서 경도 아교증과 연막밑 아교세포에서 글리코겐이 약간 증간된 양상의 경도 신경손상을 보이고, 표재층에서 신경세포의 원주조직이 파괴되며, 혈관의 확연한 증식 및 확장이 함께 관찰되었다. 그리고 100 μC/cm2/ph 이상의 전하밀도에서는 피질층 Ⅰ에서 아교증과 글리코겐이 좀 더 많이 관찰되며, 조직파편으로 채워진 큰대식세포, 세포내 치밀소체(intracellular dense bodies), 세포질 공포, 세포내 부종이 보이고, 현저한 신경세포의 수축 및 퇴화가 확인되었다. 이러한 변화는 pulse duration이나 자극시간에 따르지 않았으며, 또한 여러 연구에서도 조직학적 중증도의 차이는 흥분독성 역치에 따라 달라진다고 하였다[14,22,24,27,47].
세포자멸사와 괴사는 기전과 조직학적 변화는 다르지만 전하밀도에 따른 조직학적 변화는 두 가지 모두 겹쳐져서 나타난다(Fig. 2, Table 2). 이렇게 전기자극에 따른 세포자멸사와 괴사는 “Apoptosis-Necrosis Continuum”이라는 공유된 생화학 네트워크가 형태학적으로 표현되기도 한다[48,49].
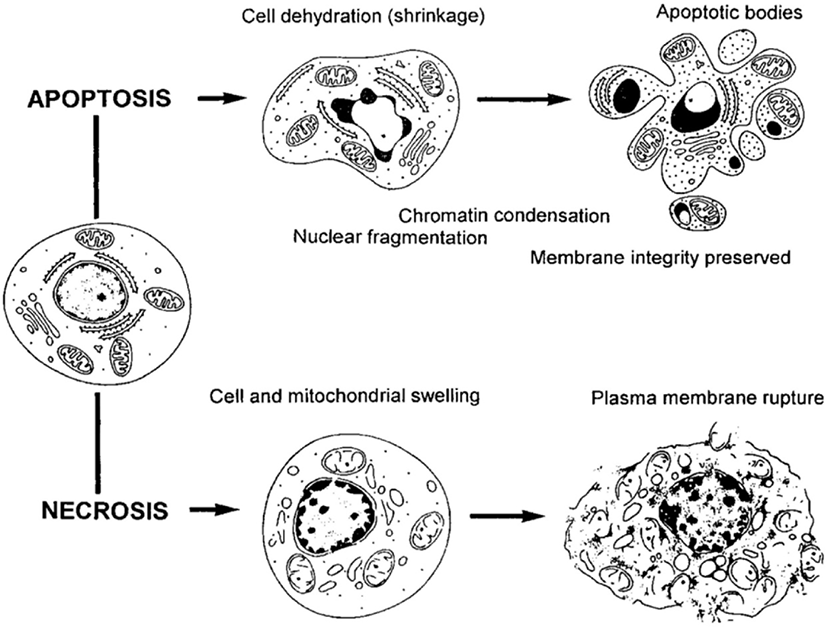
또한 고양이의 대뇌피질에 백금염(platinum salt) 혼합물을 이용한 Agnew 등의 연구에서 막 세포질 소체(membrane cytoplasmic bodies)와 Zebra 소체 등을 관찰하였다[50]. 이러한 세포질의 이상소견은 타이-삭스병(Tay-Sachs disease) 같은 효소결핍질환에서 발생하기 때문에 백금이 전기화학반응에 따라 전극이 용해 또는 부식되어 대뇌 효소 시스템에 영향을 주는 것으로 제시하였다[25,50]. 그러나 지금까지 전기자극으로 인한 막 세포질 소체나 Zebra 소체에 대한 보고는 더 이상 없다.
그런데 일부 저자는 역치를 초과한 전기자극으로 신경손상이 관찰되지만, 5–7일 이후에는 손상은 보이지 않는다고 하였다[22,24,28]. 고양이의 대뇌피질에 50 Hz의 이상성 전기자극시, Pudenz 등은 자극직후와 달리 5일 이후에만 포식활동(phagocytic activity)에 관련된 결정포함물(crystalline inclusions)이 발견된다고 하였고, McCreery 등은 자극 1주일 이후 광학현미경에서는 손상된 신경세포가 직후보다 덜 보이나, 전자현미경에서는 두드러지게 위축되고, 세포내용물이 분절화한 비가역적으로 손상된 신경세포가 관찰된다고 하였다[22,24]. 하지만 McCreery 등은 연속된 고양이의 대뇌피질연구에서 7시간 동안 50 Hz의 전기자극시, 자극 직후 손상된 것으로 보이는 많은 신경세포가 5–7일 이후에 회복될 것이라고 하였다[14]. 그리고 Oinuma 등은 40–50 mA, 500 Hz의 단극성 전기자극 시, 자극 직후의 쥐의 대뇌피질에서는 수상돌기와 사립체의 부종이 자극 직후 관찰되나, 자극 이후 30일이 된 쥐에서는 어떠한 비정상 소견이 확인되지 않는다고 보고하였다[28]. 전기자극 이후 5–7일차의 양상은 신경손상의 가역적인 회복이나 포식작용에 따른 것으로 보이며, 전기자극 시 신경손상의 자율조절(autoregulation)에 따른 결과로 생각된다.
결론
전기자극에 의한 대뇌피질 손상은 흥분독성, 전기화학반응, 열 침착 및 세포 항상성의 저하이다.
흥분독성은 전하와 전하밀도가 주요하게 작용하며, 펄스의 빈도, 기간 및 전류밀도도 영향을 미친다. 실험동물연구에서는 역치가 40 μC/cm2/ph 이상의 전하밀도이며, 일반적으로 Shannon criteria를 사용한다.
전기자극에 의한 신경손상은 조직학적으로 세포질 공포, 염색질용해, 신경세포포식(neurophagia)이며, 마지막으로 신경세포가 완전히 소실된다. 이것은 apoptosis-necrosis continuum가 특징이다.
선행연구에 따르면 인간의 전기자극기에 대한 안전한계가 설정되어 있지만, 실험동물연구에 근거한 안전한계이다 보니 직접 인간에게 적용하는 것은 어렵다. 실험동물은 오랜 시간에 걸친 지속적인 자극을 주고 검사한 데 비해, 인간에게는 비교적 짧은 기간에 간헐적으로 주어지기 때문에 어떤 특정 자극부위에서 자극시간 전체의 길이는 실험동물에 이용된 것보다 짧다[7]. 그리고 전기자극의 길이, 해부학적 특징이 실험동물과 인간 사이에서 차이가 있다. 또한 대뇌피질에 대한 신경손상을 시사하는 임상 증상 및 징후에 대한 보고는 아직 없다.
그렇지만 우리는 아직 인간 대뇌피질에서 전기자극의 영향에 대해 제한된 지식만 가지고 있으므로 우리는 전기자극에 따른 대뇌피질의 손상기전과 더 안전한 수술중대뇌피질자극기술에 대해 연구해 나가야 한다.