Introduction
Tethered cord syndrome (TCS) is a disorder characterized by the presence of a neural tube defect, in which the distal spinal cord is anchored caudally to an immobile bony structure, secondary to embryologic failure of spinal cord development, occasionally causing injury to the conus-cauda region [1]. Untethering surgery is indicated to prevent progressive neurological deficits, ranging from simple cutting of the filum terminale to removal of the complex, intermingled lipomatous tissue, which often strictly adheres to the neural structures near the conus and cauda equina nerve roots [2]. As such, intra-operative neurophysiological monitoring (IONM), comprising a combination of monitoring and mapping of the functional integrity of all lumbosacral roots, including electromyography (EMG), motor evoked potential (MEP), somatosensory evoked potential (SSEP), and bulbocavernosus reflex (BCR) with pudendal SSEP, plays a key role in detecting and preventing neurologic injuries and maximizing the clinical efficacy of untethering surgery [3,4]. However, as lower-extremity MEP reflects the integrity of both the central and peripheral nervous systems, it is impossible to differentiate between the function of the spinal cord in conus lesions and lumbosacral root damage. The clinical presentations of conus lesions of upper motor neuron (UMN) disorder and cauda equina lesions of lower motor neuron (LMN) disorder differ completely and affect the decision for treatment and further management of weakness, spasticity, deformity, and neurogenic bladder. Herein, we demonstrate the first case in which the intra-operative central motor conduction time (CMCT) was measured during untethering surgery to provide additional information about spinal cord function, distinct from peripheral motor nerve integrity.
Case Report
A 6-month-old male baby first presented to the Department of Pediatric Neurosurgery with a sacral dimple. A diagnosis of congenital bilateral hydronephrosis (Onen’s grade I) [5] was made during the perinatal period and several episodes of pyelonephritis and urosepsis occurred since the age of 5 months. When the infant was 12-months-old, the urodynamic study revealed a bladder capacity of 70 cc and post-voiding residual urine of 30 cc, with no signs of vesicourethral reflux or detrusor-sphincter dyssynergy. Spine magnetic resonance imaging showed a thin linear fatty change in the filum terminale with a thickness of 0.33 cm at the borderline conus level of L2/3 level. Although developmental milestones were normal along with the absence of asymmetric, weak leg movements or foot deformity, the pre-operative electrodiagnosis at the age of 12 months revealed left S1 radiculopathy with denervation potentials from his left abductor hallucis muscle, suggesting mild partial axonal involvement. Considering the risk of neurogenic bladder aggravation, hydronephrosis, renal failure, and paraplegia progression, untethering surgery was performed at 15-months.
During surgery, general anesthesia was induced and maintained by continuous infusion of propofol (600 mg) and remifentanil (500 μg). Controlled muscle relaxation was achieved before tracheal intubation with 11 mg of intravenous rocuronium. The total time of anesthesia was 3 h and 15 mins, and intra-operative body temperature was maintained between 36.1? and 37.1? throughout the period of anesthesia. For the usual IONM of untethering, which is performed at the lumbosacral level, transcranial MEP and spontaneous EMG were recorded from the bilateral abductor pollicis brevis, vastus medialis, tibialis anterior, gastrocnemius, abductor hallucis, and sphincter muscles to monitor each lumbosacral root. For transcranial electrical stimulation, needle electrodes were inserted into the scalp at C3 and C4 with the International 10–20 System. A train of eight pulse stimuli was applied using a commercialized transcortical stimulator with a frequency of 500 Hz and stimulus duration of 75 μs. The mean stimulation intensity was set to 600 V. The SSEP was monitored at the bilateral posterior tibial and pudendal nerves. For BCR monitoring, a pair of stimulating surface disc electrodes was attached to the dorsal side of the penis, with the cathode at the proximal side and the anode at the distal side, which were also used for pudendal SSEP stimulation. The recording needle electrodes were placed in the external anal sphincter muscles, which were also used for sphincter MEP. A train of eight pulse stimuli with a frequency of 500 Hz and stimulus duration of 75 μs was applied [6]. The mean stimulation intensity was 50 mA, which was used as a cut-off for the baseline BCR response.
CMCT was defined as the conduction time from the motor cortex to the anterior horn cell in the spinal cord. The CMCT was calculated by subtracting the peripheral motor conduction time (PMCT) from the MEP latency (Fig. 1). The PMCT was calculated by adding the distal M-wave latency (M) and F-wave latency (F), subtracting 1 ms for the re-excitation time of the motor neuron in the spinal cord, and finally dividing by two (equation (1)) [7,8]. The F-wave latency reflects the late motor response from antidromic stimulation travelling up the motor nerve to the anterior horn cell, with backfiring and orthodromic travelling back down the nerve to the muscle. The shortest of the F-wave latencies, called the minimum latency, was used for the calculation. Distal M-wave latency was defined as the onset of a compound motor action potential (CMAP), which was measured by distal motor stimulation with direct muscle response from orthodromic travel. Therefore, the formula used for estimating the CMCT is as follows (Fig. 1):
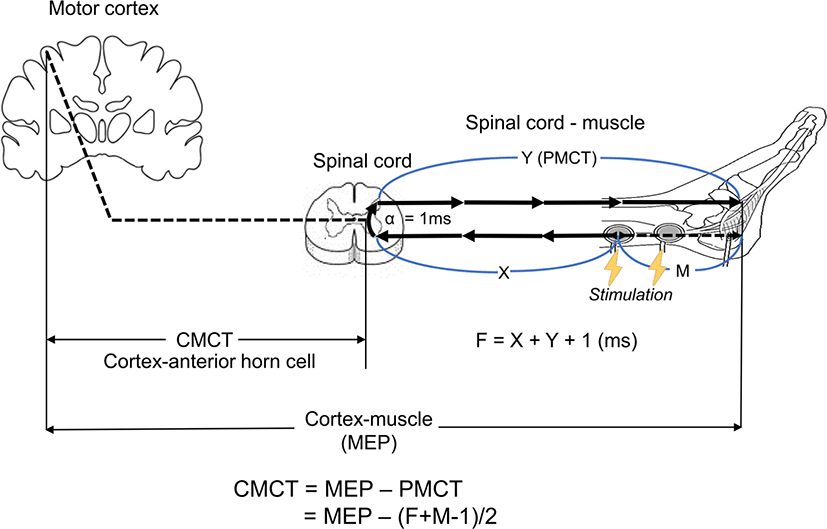
To detect CMCT during surgery, intra-operative evaluation of F- and M-wave latency in addition to MEP latency is necessary. A pair of surface disc electrodes was attached around both medial malleoli to stimulate the tibial nerves with recording needle electrodes in both abductor hallucis muscles (Fig. 2). To obtain the CMAP of the abductor hallucis, a distal surface electrode was set as the cathode, and a proximal surface electrode was set as the anode. Thereafter, the placement of the electrodes remained the same, but the direction was set in the reverse order; i.e., a distal electrode was set as the anode, and a proximal electrode was set as the cathode. It could then generate antidromic electric stimulation to obtain the minimal latency of the F-wave (Fig. 1). Supramaximal stimulation for the CMAP and F-wave was used by gradually increasing the stimulation intensity from 30 mA to ensure that the largest CMAP amplitude was obtained (at a stimulation intensity of 50–70 mA). The CMAP and F-wave were recorded in three consecutive stimulation trains (Fig. 3) to acquire acceptable potentials and improve the reliability. The filter setting was 100–1,000 Hz to remove the background noise. Then, together with routine monitoring of MEP latency of the abductor hallucis muscles, CMCT was calculated according to the formula (1) shown in Fig. 1.
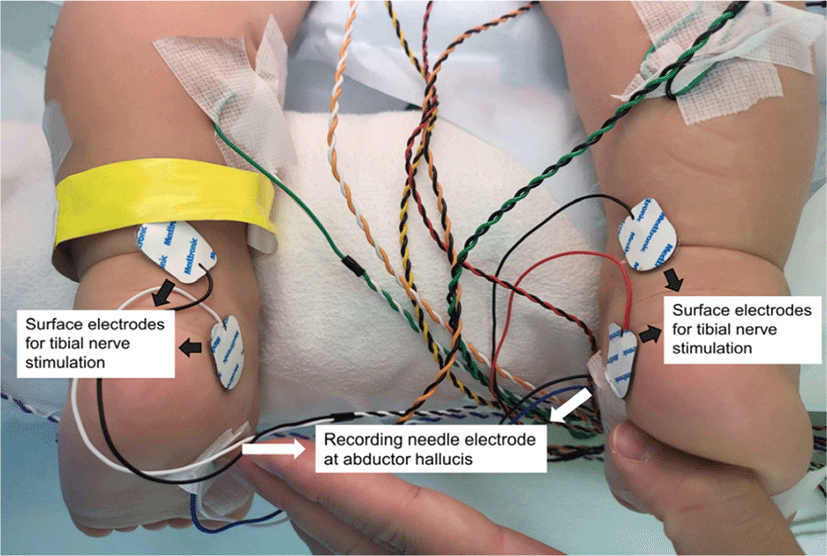
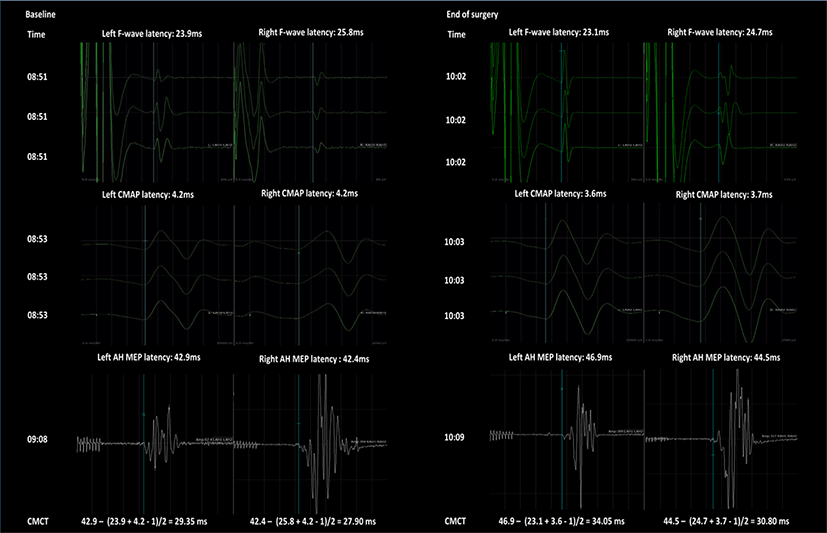
During surgery, CMCT was measured only twice to evaluate spinal cord function; before the dural incision as a baseline evaluation, and after the dural closure as a follow-up evaluation (Fig. 3). The initial M-wave latency, minimal F-wave latency, and MEP latency were 4.2 ms, 25.8 ms, and 42.4 ms on the right side and 4.2 ms, 23.9 ms, and 42.9 ms on the left side, respectively. Therefore, initial CMCT was calculated as 27.90 ms on the right side and 29.35 ms on the left side. The follow-up M-wave latency, minimal F-wave latency, and MEP latency were 3.7 ms, 24.7 ms, and 44.5 ms on the right side and 3.6 ms, 23.1 ms, and 46.9 ms on the left side, respectively. As such, the follow-up CMCT was calculated as 30.80 ms on the right side and 34.05 ms on the left side (Fig. 3). The patient’s height and weight on the day of the operation were 80 cm and 11.8 kg, respectively.
While cutting the thickened filum (1.5 mm), the triggered EMG responses from direct nerve stimulation at 20uV was utilized to demonstrate that there were no functional roots around the incision. Further, there were no significant changes in other modalities, such as MEP, SSEP, EMG, and BCR, during surgery. After surgery, there were no new neurologic deficits compared to the pre-operative neurologic or functional status.
Discussion
Herein, we report the first case in which intra- operative CMCT was measured in addition to EMG, MEP, SSEP, and BCR during an untethering surgery involving manipulation of the lumbosacral rootlets. The CMCT is defined as the time taken for the nerve impulse to travel from the primary motor cortex to the spinal motor neuron, and therefore reflects function of central nervous system, specifically that of spinal cord in this case. It is difficult to evaluate preoperative CMAP and F-waves, especially in pediatric patients, as repetitive electric stimulation is generally intolerable in children. In contrast, if the location of the stimulating surface electrodes is well fixed during surgery, the M- and F-waves from three consecutive stimulations can be easily obtained with stable minimal latencies (Fig. 3). The CMCT could be measured by adding only a pair of painless surface electrodes, while recording needle electrodes used for MEP latency are utilized concurrently (Fig. 2). Therefore, intra-operative monitoring of CMCT does not require additional specialized instruments, and can be applied whenever the spinal cord function is in question.
Most neurologic impairments in the TCS occur at the level of the conus medullaris or cauda equina, as the anchored and stretched caudal spinal cord undergoes progressive neurological injuries [9]. The CMCT in patients with TCS was longer than that in healthy controls, reflecting the common UMN lesions [10] combined with radiculopathies. Previously, lower-limb MEP was monitored during surgery to recognize injuries to the spinal cord and nerve roots altogether, but this MEP alone could not differentiate between the UMN and LMN lesions. If CMCT is measured with other modalities of IONM, changes in either CMCT or PMCT/MEP latency at the end of the operation can provide useful clinical information. When MEP latency is delayed, if CMCT does not change and PMCT prolongation occurs at the end of the surgery, LMN injuries are implicated, indicating the impairment of lumbosacral nerve roots, and vice versa.
However, it is difficult to monitor CMCT as continuously as other modalities during the operation as the stimulation direction must be reversed by switching the cathode/anode. Therefore, the clinical value of CMCT measurement is more of a localization than of monitoring nerve damage, and its evaluation is necessary only when an alarming MEP event occurs. The CMCT data can also help to determine the level of neurologic injuries from UMN to LMN lesions, helping clinicians to anticipate postoperative neurological impairment phenotypes and prepare for further management. Moreover, the CMCT data, especially those measured at the end of the surgery, representing the final spinal cord function, can be used as additional information to decide whether postoperative neurologic sequelae is re-tethering or just persistent remaining neural injuries in future follow-ups. For example, if neurogenic bladder or weakness is aggravated during follow-up, it is difficult to determine whether this is a new deterioration suggestive of re-tethering, or just a natural change in pre-existing spinal cord injuries. If the localization of former injuries is accurate, it becomes easier for a correct diagnosis and prevents unnecessary surgeries.
In this case, involvement of left more than right S1 radiculopathies and denervation potentials existed in the pre-operative electrodiagnosis. Although the patient showed no definite neurologic impairment or alarming signals from IONM, the initial CMCT on the left side was slower than that on the right side. Subsequently, CMCT at the end of the surgery showed greater left side slowing, with the difference in CMCT between the left and right sides increasing from 1.42 ms at the beginning to 3.25 ms at the end of the surgery. This suggests that clinicians should carefully examine patients with left leg weakness, as this may be more vulnerable than the right side.
This study has some limitations. First, differences between the initial and follow-up CMCT data were mostly based on MEP latency rather than on M- or F-wave latency (Fig. 3). Thus, the effects of the analgesic agent dose and anesthetic duration may affect the follow-up CMCT more at the end of surgery. However, the train of four (TOF) could not be measured because of the small body size of the child. A detailed analysis of CMCT changes during surgery should follow TOF measurements in the future. Second, as this case has not yet been evaluated for future neurologic prognosis, and there is a lack of data about the normal ranges of CMCT with respect to the height or leg lengths of different patients, there was no criterion to determine a significant CMCT change during surgery. Future studies with further evaluation of intra-operative CMCT in various spinal cord surgeries are necessary to address these limitations.
In conclusion, the intra-operative CMCT measurement included in IONM for spinal cord surgery at the lumbosacral level, a pediatric untethering surgery in this case, is a readily accessible method for evaluating and localizing conus-cauda lesions. The addition of intra- operative CMCT to previous IONM modalities can provide additional information about the spinal cord or lumbosacral nerve root impairment during surgery. As this is a novel approach in IONM, cutoff values for determining significant prolongation of CMCT during surgery should be established in future studies.